MesoAmerican Subduction Experiment (MASE)
MASE Home |
People |
Science |
Data |
Publications |
Outreach |
Images |
Relevant Links
Science - for a more technical discussion, see references listed under publications
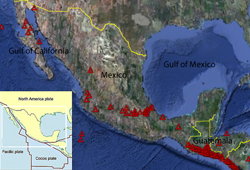
Figure 1. Deep ocean trench (dark blue) off southern shore of Mexico
|
Mexico is located on one of Earth's subduction zones, where an oceanic tectonic plate, the Cocos plate, is diving ("subducting") beneath a continental tectonic plate, the North American plate. The two tectonic plates meet off shore, forming a deep trench parallel to the shoreline (see Figure 1). This area is called the Middle American Subduction Zone.
Subduction zones, where one plate forces its way below another, are prone to earthquakes, for the motion is not smooth (see animation). They are also home to volcanic arcs located about 100 km (60 miles) inland that run parallel to the trench. These are produced by melting in the mantle below, caused by water being removed from the subducting slab as it descends beneath the continent.
Until a few years ago, this subduction zone was thought to be like others around the globe, such as Sumatra, Cascadia, and Japan. But through the work of the TO, scientists now know it is anything but "normal:"
-
In most subduction zones, the subducting slab dives down at a steep angle, nearly 45°, directly into the mantle. But in southern Mexico, just as the subducting slab is about to enter the mantle, it instead levels off to nearly horizontal, "hugging" the continental crust above it for a distance of 250 km (150 miles).
- And in most subduction zones, giant earthquakes occur every few hundred years or so. While such earthquakes do occur along the coast in this region as well, recently another form of earthquake has been discovered, called a slow slip event (or "silent earthquake"). These events occur so slowly that a single "earthquake" can last as long as a month. And the seismic waves they produce are so weak that people cannot feel them. In central Mexico, the slow slip events occur approximately every 4 years and last for 6 months (see Figure 6). They are the energy equivalent of a magnitude 7 earthquake.
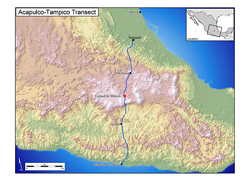
Figure 2. The MASE array of 100 seismometers crossing Mexico
|
Usually, scientists obtain an image of the subducting slab by using traditional analysis of seismic data to locate the sources (hypocenters) of earthquakes. This method works near the coast of the Mexican subduction zone; however, further inland, where the slab is known to be shallow, there are too few earthquakes.
To get around this problem, TO scientists are using a P to S converted phase in a method commonly called Receiver Function Analysis. This method locates interfaces below the surface of the earth (such as the crust/mantle boundary) by detecting how the interface affects the properties of seismic waves. (For example, the crust/mantle boundary can change a P wave into an S wave). However, it provides a very localized picture, only showing what is directly below an individual seismometer.
To see over a larger distance, TO scientists installed a dense array of 100 broad-band seismometers, cutting across Mexico and spaced 5 km apart (see Figure 2). The line, called the MASE (MesoAmerican Subduction Experiment) array, extends from Acapulco on the Pacific coast, through Mexico City, almost to Tempico on the Gulf of Mexico. This array was installed in 2005. (See installation of a seismometer)
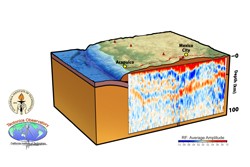
Figure 3. Receiver Function Analysis showing flat slab subduction [Modified from Perez-Campos et al., GRL 2008]
|
What TO scientists discovered from this analysis of MASE data surprised everyone. The high-resolution image of the subducting slab underneath Mexico shows the slab to be so shallow that it is actually lying flat up against the continental crust above it. And it stays this way, nearly horizontal, for 250 km, nearly all the way to Mexico City. This is very unusual. It is given the name "flat slab subduction" (see Figure 3).
In the figure, the seismometers (black triangles) run along the top, their height showing the topography. On the left is Acapulco, in the middle Mexico City and the volcanic belt, and on the right is the city of Tempoal. The volcanic arc is seen to lie further inland than expected for a subduction zone. The Receiver Functions below each station reveal the position of the subducting slab. The inset at the bottom left shows a blow-up of the flat slab region. The slab position is shown by the darker blue signal. The light blue area above is continental crust, the light orange area below is mantle. The slab lies right up against the crust.
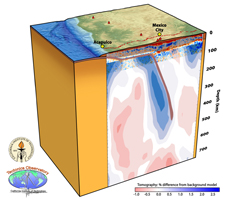
Figure 4. Full Image of Subducting Slab [From Perez-Campos et al., GRL 2008]
|
To see what happens to the slab at even greater depths, TO scientists use another method of seismic data analysis, Seismic Tomography. Though lower in resolution than Receiver Function Analysis, Seismic Tomography can be used to obtain images down to 700 km. This method detects changes in seismic wave speed due to changes in the earth's density. Waves speed up when passing through denser rock; they slow down when passing through less dense rock. Since the subducting slab is more dense than the surrounding mantle, its position can be determined.
Combining the results of both Receiver Function Analysis and Seismic Tomography, TO scientists obtained a full image of the subducting slab (see Figure 4). The contour lines indicate wave speed. Blue lines show where seismic waves traveled faster, indicating the area is dense (hence the subducting plate). Pink lines show where waves traveled slower, indicating the area is less dense (hence the mantle).
The slab (purple line) begins with a shallow dive below Acapulco, then levels out almost to Mexico City. Below Mexico City it plunges steeply into the mantle and ends abruptly at a depth of about 500 km. This abrupt termination of the plate may be due to an earlier tear at that location, before that portion of the ocean floor had subducted.
This image explains why the volcanic arc does not run parallel to the trench. In subduction zones, volcanoes are formed when the subducting plate disrupts the mantle, causing it to melt into magma. This occurs at about 100 km depth. It does this by bringing water down with it (oceanic crust is porous). The water mixes with the mantle, causing patches to melt at a lower temperature than usual. For most subduction zones, the subducting plate reaches 100 km in depth at about 100 km inland from the trench; hence most volcanic arcs are located 100 km from the trench. But in southern Mexico, because the subducting slab flattens out, it does not reach 100 km in depth until about 300 km from the trench, thus forming volcanoes 300 km from the trench. Since this flat slab subduction zone is bounded on both side by normal subduction, the line of volcanoes is not straight.
This work also provides insights into the history of subduction zones that occurred long ago. One example of this is the Farallon plate, which subducted under the western continental United States several hundred years ago. When it subsequently flattened out, it not only raised the great plains to their current mile-high elevation but also moved the coastal volcanoes inland, all the way to Kansas.
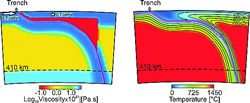
Figure 5. Model results for evolution of flat subduction [From Perez-Campos et al., GRL 2008]
|
What were the conditions that caused this subducting slab to flatten out? And what then caused it to end abruptly at 500 km depth? This is an active area of research.
TO scientists are combining theory and observations into a dynamical model to simulate the forces inside the earth over the last 22 million years. With these models, they are able to watch the evolution of a subduction zone over this time span. In particular they watch the thermal and compositional state of the mantle wedge and any melting, the shape of the slab, the coupling between the overriding
and down-going plates, and the thermal history of the upper plate.
The Central America subduction
zone is a good location to study this problem, because significant variations
in behavior and characteristics along strike are present there. These
variations do not appear to correlate with slab age or plate convergence
rate. However, they may be controlled by thermal or compositional
variations in the mantle wedge itself, by features that were riding on the
down-going plate and subsequently were subducted, or by characteristics of
the subduction interface itself. The geochemistry of the magmatic rocks in
this region has the potential to record the temperature, water content,
source region, and the type and extent of melt in the mantle wedge.
Using such models, TO scientists have found that flat slab subduction might have been caused by a layer of low-viscosity (easily flowing) material right below the crust. This layer would have given way as the subducting slab pressed against it from below. One possible source of a low-viscosity mantle layer could be the subducting plate bringing along water as it dives down. Assuming such a scenario, model results showing the resulting slab (see Figure 5) look similar to that obtained by the seismic data above. By having a low-viscosity mantle wedge (in red) just below the crust, the subducting plate can flatten as it squeezes that material out of the way.
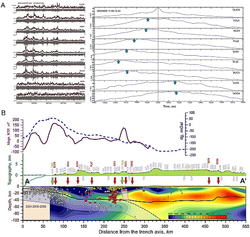
Figure 6. Detection of NVT and location of their sources [Modified from Payero et al., GRL 2008]
|
Another unusual aspect about the Mexican subduction zone is the occurrence of seismic signals so small that people cannot feel them, nor can individual seismometers detect them. It was only by using the technique of cross correlation (comparing seismographs from different instruments) that TO scientists discovered these weak signals, called "NonVolcanic Tremor (NVT)." (The qualifier "nonvolcanic" distinguishes these from tremor produced by volcanoes.)
NVT signals for nine seismometers are shown on the left-hand side of Figure 6. The corresponding cross-correlation functions are shown on the right-hand side. The blue arrows show the times that different stations detected the same seismic wave. From this analysis, the sources of these signals (called hypocenters) can be located (see stars in Panel B). They mainly occur at and above the plate boundary (blue hazy ribbon), indicating that stress in the upper plate causes them.
Could plate motion be the cause of these NonVolcanic Tremors? To answer this question, TO scientists looked at GPS (Global Positioning System) data to monitor upper plate motion during the time of the NonVolcanic Tremors. Both GPS data (yellow) showing slow slip, and seismic data (red and green) showing nonvolcanic tremors, are plotted on the same graph in Figure 7. The GPS data shows two slow earthquakes, in early 2002 and mid-2006. The clustering of nonvolcanic tremors during these two events (circled areas) confirms that slow slip is one cause of the tremor.
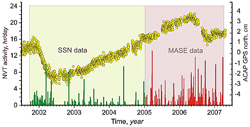
Figure 7. NonVolcanic Tremor and its relation to slow slip. [from Payero et al., GRL 2008]
|
The discovery of NonVolcanic Tremors in this region not only provides a window into the dynamics of subduction zones and the events that lead to major earthquakes, but may also someday improve earthquake hazard assessment. It is not yet clear whether there is any relation between the giant earthquakes of subduction zones and slow slip. Do the slow slip events relieve strain and thus lessen seismic hazard, or, by relieving strain in one area, do they cause strain to build up elsewhere and thus increase seismic hazard? This is an active area of research.
|